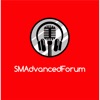
NASA Finally Contacts Voyager 2 After Unprecedented Seven-Month Silence
SMAF-NewsBot
English - November 04, 2020 09:58 - 3.51 MBTechnology News Tech News streaming industry news technology media smaf smadvancedforum Download Apple Podcasts Google Podcasts Overcast Castro Pocket Casts RSS feed
Share to Linkedin
In the history of spaceflight, only five spacecraft ever launched by humanity possess enough energy to leave the gravitational pull of our Solar System. While thousands upon thousands of objects have been launched into space, overcoming the gravitational pull of planet Earth, the Sun is more than 300,000 times as massive as our home planet, and is far more difficult to escape from. A combination of fast launch speeds and gravitational assists from other planets were required to leave our Solar System, with only Pioneer 10 and 11, Voyager 1 and 2, and New Horizons attaining “escape velocity” from our Sun.
While Pioneer 10 and 11 are now inactive, New Horizons and both Voyager spacecrafts remain operational, powered by radioisotope thermoelectric generators. Voyager 1 has overtaken all other spacecrafts and is now the most distant: 22 billion km away, pulling away from the slightly slower Voyager 2 at “only” 18.8 billion km distant. Since the coronavirus pandemic in mid-March, NASA has had no contact with Voyager 2, but an upgraded deep space network dish made a successful call on October 29. Here’s the fascinating science that keeps us in touch with the most distant objects ever launched from Earth.
When it comes to sending and receiving signals across astronomical distances, there are three enemies you have to overcome:
1. distance,
2. time,
3. and power.
Recommended For You
This Week Jupiter Aligns With Saturn. What Happens Next Will Be A Once-In-A-Lifetime Sky Event
Did A Black Hole Give Birth To Our Universe?
### Your Stargazing Guide To November: A Comet, A ‘Frosty Moon Eclipse’ And A Chance Of Fireballs
The farther away a spacecraft is from you, the farther a signal that you send has to travel before it reaches it, the longer it takes to get there, and the lower in power that signal is when it arrives. If a spacecraft is twice as distant as another, the distance to it is twice as great, the time it takes a light signal to travel to it is twice as great, and the signal power that it receives is only one-fourth as great, since light signals spread out in the two dimensions perpendicular to the spacecraft’s line-of-sight. The farther away a spacecraft is, it’s harder to contact, it takes longer to contact it, and it requires more energy to send-or-receive the same signal.
The way an electromagnetic signal works — whether you’re detecting it with a refracting lens, a reflecting dish, or a linear antenna — is straightforward: it spreads out in a spherical shape from its source. Because there’s a certain amount of inherent background noise to any observation you’d make, from both terrestrial and celestial sources, you need your signal to cross a certain threshold to be detectable, rising above the noise background. On the receiving end, that means larger detectors are better, while on the transmitting end, that means a higher-powered transmitter is better.
Unfortunately, the spacecraft that have already been launched cannot have their hardware upgraded in any way; once they’re launched, they’re simply stuck with the technology they’ve been outfitted with. To make matters worse, the spacecraft themselves are powered by radioactive sources, where specially chosen material, such as plutonium-238, radioactively decays, emitting heat that gets converted into electricity. As time goes on, more and more of the material decays away, decreasing the power available to the spacecraft for both transmitting and receiving signals.
As the amount of heat energy produced by radioactive material decreases, the conversion from heat energy into electrical energy becomes less successful: the thermocouples degrade over time and lose efficiency at lower powers. As a result, the power available to the spacecraft through radioisotope thermoelectric generators has decreased precipitously. As of 2020, the plutonium-238 onboard is producing just 69% of the initial heat energy, and that translates into only ...
Share to Linkedin
In the history of spaceflight, only five spacecraft ever launched by humanity possess enough energy to leave the gravitational pull of our Solar System. While thousands upon thousands of objects have been launched into space, overcoming the gravitational pull of planet Earth, the Sun is more than 300,000 times as massive as our home planet, and is far more difficult to escape from. A combination of fast launch speeds and gravitational assists from other planets were required to leave our Solar System, with only Pioneer 10 and 11, Voyager 1 and 2, and New Horizons attaining “escape velocity” from our Sun.
While Pioneer 10 and 11 are now inactive, New Horizons and both Voyager spacecrafts remain operational, powered by radioisotope thermoelectric generators. Voyager 1 has overtaken all other spacecrafts and is now the most distant: 22 billion km away, pulling away from the slightly slower Voyager 2 at “only” 18.8 billion km distant. Since the coronavirus pandemic in mid-March, NASA has had no contact with Voyager 2, but an upgraded deep space network dish made a successful call on October 29. Here’s the fascinating science that keeps us in touch with the most distant objects ever launched from Earth.
When it comes to sending and receiving signals across astronomical distances, there are three enemies you have to overcome:
1. distance,
2. time,
3. and power.
Recommended For You
This Week Jupiter Aligns With Saturn. What Happens Next Will Be A Once-In-A-Lifetime Sky Event
Did A Black Hole Give Birth To Our Universe?
### Your Stargazing Guide To November: A Comet, A ‘Frosty Moon Eclipse’ And A Chance Of Fireballs
The farther away a spacecraft is from you, the farther a signal that you send has to travel before it reaches it, the longer it takes to get there, and the lower in power that signal is when it arrives. If a spacecraft is twice as distant as another, the distance to it is twice as great, the time it takes a light signal to travel to it is twice as great, and the signal power that it receives is only one-fourth as great, since light signals spread out in the two dimensions perpendicular to the spacecraft’s line-of-sight. The farther away a spacecraft is, it’s harder to contact, it takes longer to contact it, and it requires more energy to send-or-receive the same signal.
The way an electromagnetic signal works — whether you’re detecting it with a refracting lens, a reflecting dish, or a linear antenna — is straightforward: it spreads out in a spherical shape from its source. Because there’s a certain amount of inherent background noise to any observation you’d make, from both terrestrial and celestial sources, you need your signal to cross a certain threshold to be detectable, rising above the noise background. On the receiving end, that means larger detectors are better, while on the transmitting end, that means a higher-powered transmitter is better.
Unfortunately, the spacecraft that have already been launched cannot have their hardware upgraded in any way; once they’re launched, they’re simply stuck with the technology they’ve been outfitted with. To make matters worse, the spacecraft themselves are powered by radioactive sources, where specially chosen material, such as plutonium-238, radioactively decays, emitting heat that gets converted into electricity. As time goes on, more and more of the material decays away, decreasing the power available to the spacecraft for both transmitting and receiving signals.
As the amount of heat energy produced by radioactive material decreases, the conversion from heat energy into electrical energy becomes less successful: the thermocouples degrade over time and lose efficiency at lower powers. As a result, the power available to the spacecraft through radioisotope thermoelectric generators has decreased precipitously. As of 2020, the plutonium-238 onboard is producing just 69% of the initial heat energy, and that translates into only ...